Directly Imaging Exoplanets
Currently, I work on the Gemini Planet Imager Exoplanet Survey (GPIES) team. This very exciting project is one of two major ongoing initiatives (together with the European SPHERE Project) designed to push the limits of direct imaging of giant planets to solar-system scales.
We have only been in full science operation since late 2014, but we were thrilled to announce the discovery of the Jupiter-like exoplanet 51 Eridani b in August, 2015.
The Gemini Planet Imager is an “Integral Field Spectrograph” which means that we take images of a planet at a number of different wavelengths of light simultaneously, creating a “spectrum” of the planet that can tell us something about it’s composition. In the case of 51 Eri b, it is faint at wavelengths of light where methane absorbs strongly, indicating that, like Jupiter, the planet has methane in its atmosphere. You can see this in the animation below. The planet, indicated by the white arrow, is easily visible at some wavelengths, and then disappears at wavelengths that correspond to methane absorption.
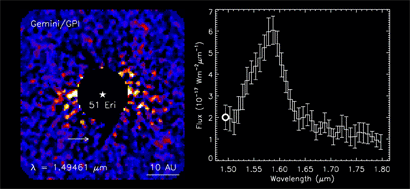
Direct Imaging of exoplanets with AO systems, like all technologies, is subject to selection effects and is best at certain kinds of problems. In the case of direct imaging, selection effects mean that we are best able to image very large planets (many Jupiter masses) that are very distant from their stars. This makes sense, because bigger planets are brighter, and the farther away they are from their host star, the easier it is to separate their light. However, thanks to ever advancing AO and imaging technologies, we are pushing to smaller and smaller planets closer to their host stars, and therefore moving toward imaging true solar system analogs. The next generation of space telescopes may be able to push this technology even further and image Earth-analogs around other stars.
Holey Disks: Illuminating the Planet Formation Process
I am also involved in imaging circumstellar disks with the Gemini Planet Imager and as a member of the Strategic Exploration of Exoplanets and Disks with Subaru (SEEDS) campaign. In both cases, I’m particularly interested in a subclass called transitional disks, which have large cleared holes at their centers. One of the most compelling explanations for why these holes exist is that planets forming in the disk have swept up the material in the inner region. I devoted much of my dissertation work to studying whether planet formation is the most viable of several competing theories for how these gaps are formed.
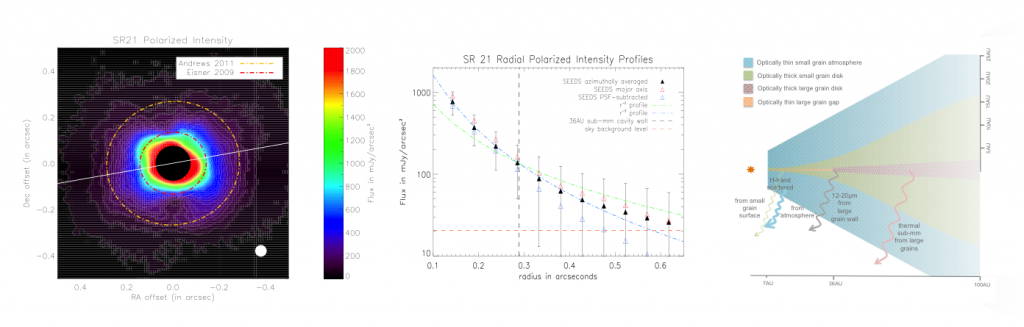
In 2013, I published a paper on SEEDS data of the disk SR21 showing that transitional disk cavities can appear very different at different wavelengths. SR21 was identified as a transitional disk because as viewed in radio light, it has a large, very empty cavity. However, my near-infrared adaptive optics images of the disk showed no such clearing (to my great initial disappointment). In fact, the brightness of the disk in the near-infrared decreases smoothly from the star to the outer disk edge, without any indication that anything special is happening at the cavity radius. It makes for a somewhat boring image, but a very exciting explanation; it turns out that this phenomenon (called “dust filtration”), where large dust grains that emit at radio wavelengths are cleared out of the disk cavity and small grains are relatively unaffected, was a prediction of planet formation theories.
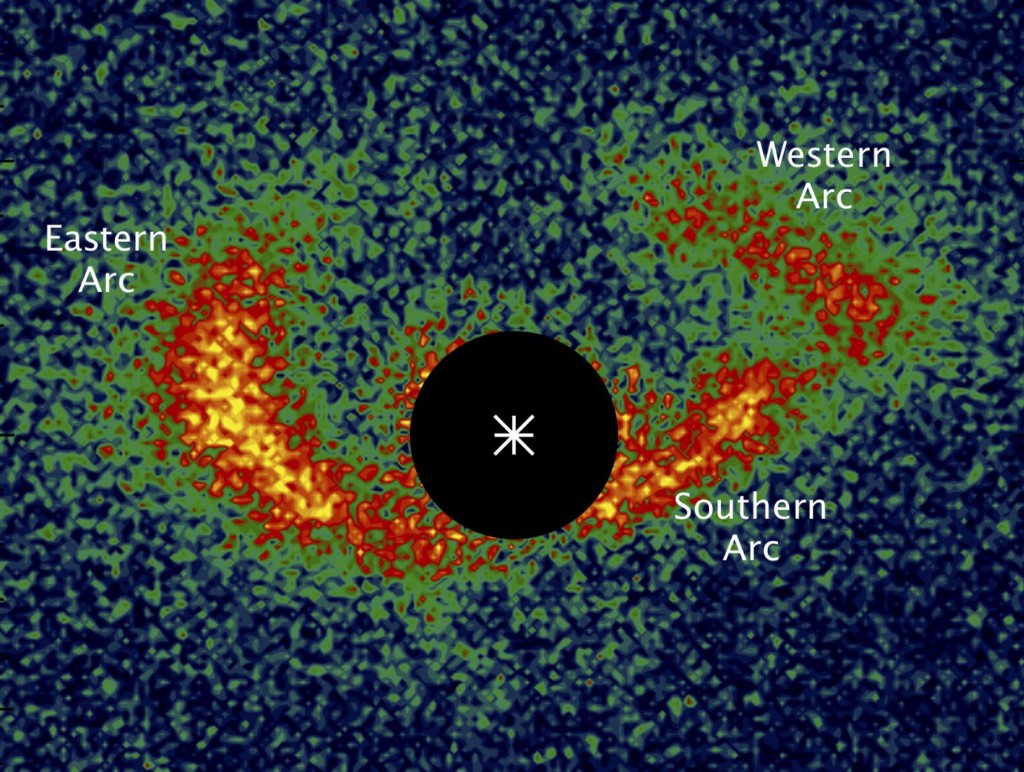
In 2015, I published a second paper using SEEDS transitional disk data, this time on the disk around the young star Oph IRS 48. This transition disk was the subject of a Science paper in 2014 showing that it had a whopping radio excess concentrated to the south of the star, just outside of the cavity radius. The authors hypothesized that this was a result of the gravitational influence of one or more planets that lie inside the disk cavity. My infrared images showed a similarly strange and very asymmetrical disk. In particular, they show that the cavity rim is very non-circular. It seems that planet formation, which is easily able to reproduce the asymmetries in the disk at both near-infrared and radio wavelengths, is the most viable explanation for this disk as well.
Accreting Protoplanets: Catching Planets in the Act of Formation
During my graduate work at the University of Arizona, I helped to commission the Magellan Adaptive Optics system (MagAO), the first AO system on a large telescope capable of visible light imaging. See our group website for more information about this very exciting project, including press about our first light results and a brief summary of my first light paper (Follette et al. 2013B).
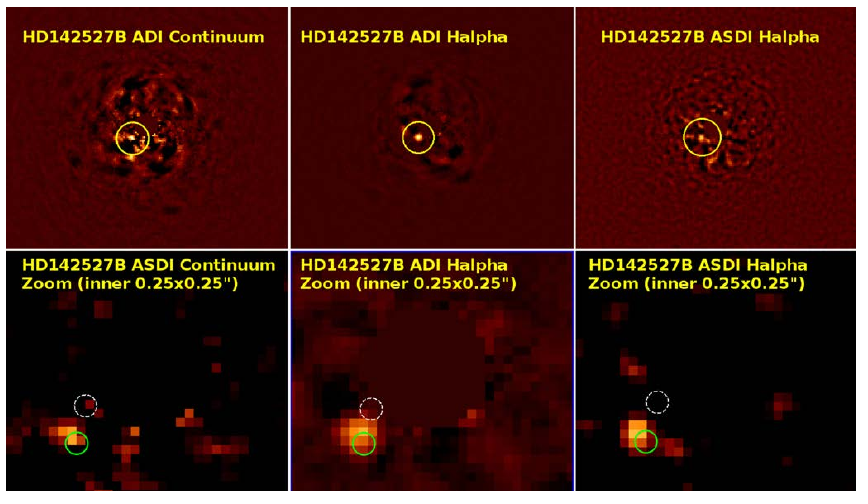
During my last year as a graduate student, I began the Giant Accreting Protoplanet Survey (GAPplanetS), which takes advantage of the visible light capabilities of MagAO to image planets that are in the process of forming inside transition disk gaps. In order to do this, I employ several clever tricks. First, by imaging at visible wavelengths, I’m able to probe the disks at very high resolution (because the resolution of an image is proportional to wavelengths, so small wavelengths mean imaging on very small scales). Secondly, I image each disk simultaneously at two visible wavelengths – one of them a wavelength where accreting objects are very bright (an emission line of Hydrogen called H-alpha), and another nearby “continuum” wavelength where nothing interesting is happening. The idea is that a star should be the same brightness at both wavelengths, while the planet will be significantly brighter at H-alpha. If we subtract the continuum from the H-alpha image, accreting objects (like forming protoplanets) stick out like a sore thumb.
Indeed, the first transition disk we looked at for GAPplanetS contained just such a source, with an accreting companion at just 86mas. The existence of this companion, which had been found using interferometric data before, was controversial until we imaged it directly. Although the mass of the object is still up for debate, it appears that it is most likely a small accreting star. The large mass of this companion may help to explain some of the strange things about this transitional disk, including its very large and very empty cavity and the fact that gas seems to be streaming into the cavity at a very high velocity.